Biology - Karl Irvin Baguio (top rated books of all time .txt) 📗
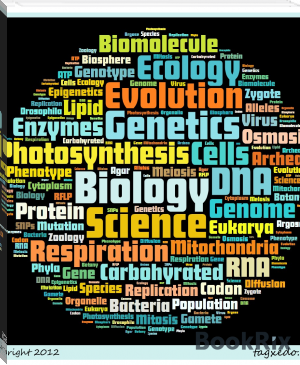
- Author: Karl Irvin Baguio
Book online «Biology - Karl Irvin Baguio (top rated books of all time .txt) 📗». Author Karl Irvin Baguio
Many enzymes are assisted by chemical substances called cofactors. Cofactors may be ions or molecules associated with an enzyme and are required in order for a chemical reaction to take place. Ions that might operate as cofactors include those of iron, manganese, and zinc. Organic molecules acting as cofactors are referred to as coenzymes.
Adenosine Triphosphate (ATP)
The chemical substance that serves as the currency of energy in a cell is adenosine triphosphate (ATP). ATP is referred to as currency because it can be “spent” in order to make chemical reactions occur. The more energy required for a chemical reaction, the more ATP molecules must be spent.
Virtually all forms of life use ATP, a nearly universal molecule of energy transfer. The energy released during catabolic reactions is stored in ATP molecules. In addition, the energy trapped in anabolic reactions (such as photosynthesis) is trapped in ATP molecules.
An ATP molecule consists of three parts. One part is a double ring of carbon and nitrogen atoms called adenine. Attached to the adenine molecule is a small five-carbon carbohydrate called ribose. Attached to the ribose molecule are three phosphate units linked together by covalent bonds.
The covalent bonds that unite the phosphate units in ATP are high-energy bonds. When an ATP molecule is broken down by an enzyme, the third (terminal) phosphate unit is released as a phosphate group, which is an ion. When this happens, approximately 7.3 kilocalories of energy are released. (A kilocalorie equals 1,000 calories.) This energy is made available to do the work of the cell.
The adenosine triphosphatase enzyme accomplishes the breakdown of an ATP molecule. The products of ATP breakdown are adenosine diphosphate (ADP) and a phosphate ion. Adenosine diphosphate and the phosphate ion can be reconstituted to form ATP, much like a battery can be recharged. To accomplish this, synthesis energy must be available. This energy can be made available in the cell through two extremely important processes: photosynthesis.
ATP Production
ATP is generated from ADP and phosphate ions by a complex set of processes occurring in the cell. These processes depend on the activities of a special group of coenzymes. Three important coenzymes are nicotinamide adenine dinucleotide (NAD), nicotinamide adenine dinucleotide phosphate (NADP), and flavin adenine dinucleotide (FAD).
NAD and NADP are structurally similar to ATP. Both molecules have a nitrogen-containing ring called nicotinic acid, which is the chemically active part of the coenzymes. In FAD, the chemically active portion is the flavin group. The vitamin riboflavin is used in the body to produce this flavin group.
All coenzymes perform essentially the same work. During the chemical reactions of metabolism, coenzymes accept electrons and pass them on to other coenzymes or other molecules. The removal of electrons or protons from a coenzyme is oxidation. The addition of electrons to a molecule is reduction. Therefore, the chemical reactions performed by coenzymes are called oxidation-reduction reactions.
The oxidation-reduction reactions performed by the coenzymes and other molecules are essential to the energy metabolism of the cell. Other molecules participating in this energy reaction are called cytochromes. Together with the coenzymes, cytochromes accept and release electrons in a system called the electron transport system. The passage of energy-rich electrons among cytochromes and coenzymes drains the energy from the electrons to form ATP from ADP and phosphate ions.
The actual formation of ATP molecules requires a complex process called chemiosmosis. Chemiosmosis involves the creation of a steep proton (hydrogen ion) gradient. This gradient occurs between the membrane-bound compartments of the mitochondria of all cells and the chloroplasts of plant cells. A gradient is formed when large numbers of protons (hydrogen ions) are pumped into the membrane-bound compartments of the mitochondria. The protons build up dramatically within the compartment, finally reaching an enormous number. The energy released from the electrons during the electron transport system pumps the protons.
After large numbers of protons have gathered within the compartments of mitochondria and chloroplasts, they suddenly reverse their directions and escape back across the membranes and out of the compartments. The escaping protons release their energy in this motion. This energy is used by enzymes to unite ADP with phosphate ions to form ATP. The energy is trapped in the high-energy bond of ATP by this process, and the ATP molecules are made available to perform cell work. The movement of protons is chemiosmosis because it is a movement of chemicals (in this case, protons) across a semipermeable membrane. Because chemiosmosis occurs in mitochondria and chloroplasts, these organelles play an essential role in the cell’s energy metabolism. Chapter 5 explains how energy is trapped in the chloroplasts in plants, while Chapter 6 explains how energy is released in the mitochondria of plant and animal cells.
Chapter 5: PhotosynthesisPhotosynthesis
A great variety of living things on Earth, including all green plants, synthesize their foods from simple molecules, such as carbon dioxide and water. For this process, the organisms require energy, and that energy is derived from sunlight.
Figure 5-1 shows the energy relationships in living cells. Light energy is captured in the chloroplast of plant cells and used to synthesize glucose molecules, shown as C6H12O6. In the process, oxygen (O2) is released as a waste product. The glucose and oxygen are then used in the mitochondrion of the plant cell, and the energy is released and used to fuel the synthesis of ATP from ADP and P. In the reaction, CO2 and water are released in the mitochondrion to be reused in photosynthesis in the chloroplast.
Figure 5-1 Energy relationships in living cells.
The process of utilizing energy to synthesize carbohydrate molecules is called photosynthesis. Photosynthesis is actually two separate processes. in the first process, energy-rich electrons flow through a series of coenzymes and other molecules. This electron energy is trapped. During the trapping process, adenosine triphosphate (ATP) molecules and molecules of nicotinamide adenine dinucleotide phosphate hydrogen (NADPH) are formed. Both ATP and NADPH are rich in energy. These molecules are used in the second process, where carbon dioxide molecules are bound into carbohydrates too form organic substances such as glucose.
Chloroplast
The organelle in which photosynthesis occurs (in the leaves and green stems of plants, for example) is called the chloroplast. Chloroplasts are relatively large organelles, containing a watery, protein-rich fluid called stroma. The stroma contains many small structures composed of membranes that resemble stacks of coins. Each stack is a granum (the plural form is grana). Each membrane in the stack is a thylakoid. Within the thylakoid membranes of the granum, many of the reactions of photosynthesis take place. The thylakoids are somewhat similar to the cristae of mitochondria (see Chapter 6).
Photosystems
Pigment molecules organized into photosystems capture sunlight in the chloroplast. Photosystems are clusters of light-absorbing pigments with some associated molecules—proton (hydrogen ion) pumps, enzymes, coenzymes, and cytochromes (see Chapter 4). Each photosystem contains about 200 molecules of a green pigment called chlorophyll and about 50 molecules of another family of pigments called carotenoids. In the reaction center of the photosystem, the energy of sunlight is converted to chemical energy. The center is sometimes called a light-harvesting antenna.
There are two photosystems within the thylakoid membranes, designated photosystem I and photosystem II. The reaction centers of these photosystems are P700 and P680, respectively. The energy captured in these reaction centers drives chemiosmosis, and the energy of chemiosmosis stimulates ATP production in the chloroplasts.
Process of Photosynthesis
The process of photosynthesis is conveniently divided into two parts: the energy-fixing reaction (also called the light reaction) and the carbon-fixing reaction (also called the light-independent reaction or the dark reaction).
Energy-fixing reaction
The energy-fixing reaction of photosynthesis begins when light is absorbed in photosystem II in the thylakoid membranes. The energy of the sunlight, captured in the P680 reaction center, causes the electrons from P680’s chlorophyll to move to a higher, unstable energy level. These electrons pass through a series of cytochromes in the nearby electron-transport system.
After passing through the electron transport system, the energy-rich electrons eventually enter photosystem-I. Some of the energy of the electron is used to pump protons across the thylakoid membrane, and this pumping sets up the potential for chemiosmosis.
The spent electrons from P680 enter the P700 reaction center in photosystem I. Sunlight activates the electrons, which receive a second boost out of the chlorophyll molecules. There they reach a high energy level. The electrons progress through a second electron transport system, but this time there is no proton pumping. Rather, the energy reduces NADP. This reduction occurs as two electrons join NADP and energize the molecule. Because NADP acquires two negatively charged electrons, it attracts two positively charged protons to balance the charges. Consequently, the NADP molecule is reduced to NADPH, a molecule that contains much energy.
Because electrons have flowed out of the P680 reaction center, the chlorophyll molecules are left without a certain number of electrons. Electrons secured from water molecules replace these electrons. Each split water molecule releases two electrons that enter the chlorophyll molecules to replace those lost. The split water molecules also release two protons that enter the cytoplasm near the thylakoid and are available to increase the chemiosmotic gradient.
The third product of the split water molecules is oxygen. Two oxygen atoms combine with one another to form molecular oxygen (O2), which is given off as the by-product of photosynthesis; it fills the atmosphere and is used by all oxygen-requiring organisms, including plant and animal cells.
Described above are the noncyclic energy-fixing reactions (see Figure 5-2). Certain plants and autotrophic prokaryotes are also known to participate in cyclic energy-fixing reactions. These reactions involve only photosystem I and the P700 reaction center. Excited electrons leave the reaction center, pass through coenzymes of the electron transport system, and follow a special pathway back to P700. Each electron powers the proton pump and encourages the transport of a proton across the thylakoid membrane. This process enriches the proton gradient and eventually leads to the generation of ATP.
Figure 5-2 The energy-fixing reactions of photosynthesis.
ATP production in the energy-fixing reactions of photosynthesis occurs by the process of chemiosmosis (explained in Chapter 4). Essentially, this process consists of a rush of protons across a membrane (the thylakoid membrane, in this case), accompanied by the synthesis of ATP molecules. Biochemists have calculated that the proton concentration on one side of the thylakoid is 10,000 times that of the opposite side of the membrane.
In photosynthesis, the protons pass back across the membranes through channels lying alongside sites where enzymes are located. As the protons pass through the channels, the energy of the protons is released to form high-energy ATP bonds. ATP is formed in the energy-fixing reactions along with the NADPH formed in the main reactions. Both ATP and NADPH provide the energy necessary for the synthesis of carbohydrates that occurs in the second major set of events in photosynthesis.
Carbon-fixing reaction
Glucose and other carbohydrates are synthesized in the carbon-fixing reaction of photosynthesis, often called the Calvin cycle after Melvin Calvin, who performed much of the biochemical research (see Figure 5-3). This phase of photosynthesis occurs in the stroma of the plant cell.
Figure 5-3 A carbon-fixing reaction, also called the Calvin cycle.
In the carbon-fixing reaction, an essential material is carbon dioxide, which is obtained from the atmosphere. The carbon dioxide is attached to a five-carbon compound called ribulose bisphosphate. Ribulose
Comments (0)