Helgoland by Rovelli, Erica (ebook reader below 3000 .txt) 📗
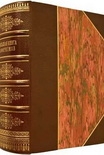
Book online «Helgoland by Rovelli, Erica (ebook reader below 3000 .txt) 📗». Author Rovelli, Erica
So what is it exactly that is so bizarre about quantum phenomena? The fact that electrons stay within certain orbits, and jump between them, is surely not the end of the world . . .
The phenomenon from which the strangeness of quanta derives is called “quantum superposition.” A quantum superposition is when two contradictory properties are, in a certain sense, present together. An object could be here but at the same time elsewhere. It is what Heisenberg means by saying that “the electron no longer has a trajectory”: the electron is found not to be only in one place or another. In a sense it is in both places. In the jargon, we say that an object can be in a “superposition” of positions. Dirac called this bizarre behavior the “principle of superposition.” For him it was the theory’s conceptual foundation.
But we need to be careful here: we never see a quantum superposition. What we see are consequences of the superposition. These consequences are called “quantum interference.” It is the interference that we see, not the superposition.
Let’s see how this works.
Quite a long time after I had studied it in books, I witnessed quantum interference with my own eyes. It was in the Innsbruck laboratory of Anton Zeilinger, a very kind Austrian with a big beard and the demeanor of a gentle bear—and one of the great experimental physicists who works wonders with quanta. He has been a pioneer of quantum computing, quantum cryptography and quantum teleportation. I’ll describe what I saw in his lab, even if subtle, because it encapsulates why physicists are confused.
Anton showed me a table with a number of optical instruments set up on it: a small laser, lenses, prism mirrors which separate the laser beam and then integrate it again, photon detectors and so on. A weak laser beam made up of a small number of photons is split into two parts, creating two separate paths—one, let’s say, on the “right,” and the other one on the “left.” The two paths are reunited before becoming separated again and ending up in two detectors: one, let’s say, “up” and the other “down.”
A beam of photons is separated into two by a prism, reunited and then divided again.
What I saw is this: If I blocked either one of the two paths (left or right) with my hand, half of the photons ended up in the down detector and half ended up in the up one (the two drawings on the left below). But if I left both paths open, free of any impediment, all of the photons ended up in the lower detector: none in the one above it (the drawing on the right below).
Try to ask yourself how this can happen.
There is something very peculiar going on. If half of the photons arrive at the up detector when one path is free, it would seem reasonable to expect that half of the photons should also arrive above when both paths are free. But this is not the case. In fact, none do.
How, by blocking one path, can my hand cause the photons traveling on the other path to go to the upper detector?
The disappearance of the photons from the upper detector when both paths are open is an example of quantum interference. There is interference between the two paths—the one on the left and the one on the right. When both are open, something happens that does not occur when photons pass on just one or the other of these paths: the photons going to the upper detector vanish.
Quantum interference. If you block one of the two paths with your hand, half of the photons arrive in the up detector (left-hand image). If the two paths are unobstructed, all the photons end up in the down one (right-hand image). How does placing my hand in one of the paths cause photons that were traveling on the other path to move to the up detector? Nobody knows.
According to Schrödinger’s theory, the ψ wave of each photon separates into two parts: two wavelets. One wavelet follows the path on the right, one takes to the left. When they meet again, the ψ wave recomposes and takes the lower path. If I block one of the paths, the ψ wave does not recompose, and therefore behaves in a different way. That waves behave in this way is not strange: interference between waves is a well-known phenomenon. Light waves and ocean waves do the same things.
But we never see the wave; we always see individual photons each passing on only one side: either to the right or to the left. If we place photon detectors along the paths, these detectors never reveal “half photon.” Each photon passes (entirely) on the left, or (entirely) on the right. Each photon behaves as if it passed through both trajectories, as waves do (otherwise there would not be interference), but if we look to see where the photon is, we always see it on just one path.
This is the quantum superposition: the photon passes “both right and left.” It is in a quantum superposition of two configurations: the one on the right and the one on the left. The consequence is the fact that the photon no longer goes upward, as it would if it passed along just one or the other of the two paths.
This is not all. There is something else, and it is truly challenging. If I measure which of the two paths the photon takes, the interference disappears!
The very act of measuring which path the photon takes causes the interference to disappear! If we measure where they pass, half of the photons go upward again.
It seems that you need only to observe what is happening for it to
Comments (0)