The Story of the Heavens - Sir Robert Stawell Ball (ebook reader for laptop .TXT) 📗
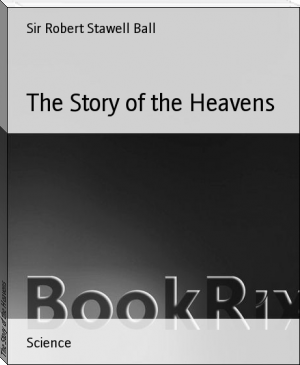
- Author: Sir Robert Stawell Ball
Book online «The Story of the Heavens - Sir Robert Stawell Ball (ebook reader for laptop .TXT) 📗». Author Sir Robert Stawell Ball
attempt to render it such justice as may be compatible with our limits.
The curve which represents with perfect fidelity the movements of a planet in its revolution around the sun belongs to that well-known group of curves which mathematicians describe as the conic sections. The particular form of conic section which denotes the orbit of a planet is known by the name of the _ellipse_: it is spoken of somewhat less accurately as an oval. The ellipse is a curve which can be readily constructed. There is no simpler method of doing so than that which is familiar to draughtsmen, and which we shall here briefly describe.
We represent on the next page (Fig. 37) two pins passing through a sheet of paper. A loop of twine passes over the two pins in the manner here indicated, and is stretched by the point of a pencil. With a little care the pencil can be guided so as to keep the string stretched, and its point will then describe a curve completely round the pins, returning to the point from which it started. We thus produce that celebrated geometrical figure which is called an ellipse.
It will be instructive to draw a number of ellipses, varying in each case the circumstances under which they are formed. If, for instance, the pins remain placed as before, while the length of the loop is increased, so that the pencil is farther away from the pins, then it will be observed that the ellipse has lost some of its elongation, and approaches more closely to a circle. On the other hand, if the length of the cord in the loop be lessened, while the pins remain as before, the ellipse will be found more oval, or, as a mathematician would say, its _eccentricity_ is increased. It is also useful to study the changes which the form of the ellipse undergoes when one of the pins is altered, while the length of the loop remains unchanged. If the two pins be brought nearer together the eccentricity will decrease, and the ellipse will approximate more closely to the shape of a circle. If the pins be separated more widely the eccentricity of the ellipse will be increased. That the circle is an extreme form of ellipse will be evident, if we suppose the two pins to draw in so close together that they become coincident; the point will then simply trace out a circle as the pencil moves round the figure.
The points marked by the pins obviously possess very remarkable relations with respect to the curve. Each one is called a _focus_, and an ellipse can only have one pair of foci. In other words, there is but a single pair of positions possible for the two pins, when an ellipse of specified size, shape, and position is to be constructed.
The ellipse differs principally from a circle in the circumstance that it possesses variety of form. We can have large and small ellipses just as we can have large and small circles, but we can also have ellipses of greater or less eccentricity. If the ellipse has not the perfect simplicity of the circle it has, at least, the charm of variety which the circle has not. The oval curve has also the beauty derived from an outline of perfect grace and an association with ennobling conceptions.
The ancient geometricians had studied the ellipse: they had noticed its foci; they were acquainted with its geometrical relations; and thus Kepler was familiar with the ellipse at the time when he undertook his celebrated researches on the movements of the planets. He had found, as we have already indicated, that the movements of the planets could not be reconciled with circular orbits. What shape of orbit should next be tried? The ellipse was ready to hand, its properties were known, and the comparison could be made; memorable, indeed, was the consequence of this comparison. Kepler found that the movement of the planets could be explained, by supposing that the path in which each one revolved was an ellipse. This in itself was a discovery of the most commanding importance. On the one hand it reduced to order the movements of the great globes which circulate round the sun; while on the other, it took that beautiful class of curves which had exercised the geometrical talents of the ancients, and assigned to them the dignity of defining the highways of the universe.
But we have as yet only partly enunciated the first discovery of Kepler. We have seen that a planet revolves in an ellipse around the sun, and that the sun is, therefore, at some point in the interior of the ellipse--but at what point? Interesting, indeed, is the answer to this question. We have pointed out how the foci possess a geometrical significance which no other points enjoy. Kepler showed that the sun must be situated in one of the foci of the ellipse in which each planet revolves. We thus enunciate the first law of planetary motion in the following words:--
_Each planet revolves around the sun in an elliptic path, having
the sun at one of the foci._
We are now enabled to form a clear picture of the orbits of the planets, be they ever so numerous, as they revolve around the sun. In the first place, we observe that the ellipse is a plane curve; that is to say, each planet must, in the course of its long journey, confine its movements to one plane. Each planet has thus a certain plane appropriated to it. It is true that all these planes are very nearly coincident, at least in so far as the great planets are concerned; but still they are distinct, and the only feature in which they all agree is that each one of them passes through the sun. All the elliptic orbits of the planets have one focus in common, and that focus lies at the centre of the sun.
It is well to illustrate this remarkable law by considering the circumstances of two or three different planets. Take first the case of the earth, the path of which, though really an ellipse, is very nearly circular. In fact, if it were drawn accurately to scale on a sheet of paper, the difference between the elliptic orbit and the circle would hardly be detected without careful measurement. In the case of Venus the ellipse is still more nearly a circle, and the two foci of the ellipse are very nearly coincident with the centre of the circle. On the other hand, in the case of Mercury, we have an ellipse which departs from the circle to a very marked extent, while in the orbits of some of the minor planets the eccentricity is still greater. It is extremely remarkable that every planet, no matter how far from the sun, should be found to move in an ellipse of some shape or other. We shall presently show that necessity compels each planet to pursue an elliptic path, and that no other form of path is possible.
Started on its elliptic path, the planet pursues its stately course, and after a certain duration, known as the _periodic time_, regains the position from which its departure was taken. Again the planet traces out anew the same elliptic path, and thus, revolution after revolution, an identical track is traversed around the sun. Let us now attempt to follow the body in its course, and observe the history of its motion during the time requisite for the completion of one of its circuits. The dimensions of a planetary orbit are so stupendous that the planet must run its course very rapidly in order to finish the journey within the allotted time. The earth, as we have already seen, has to move eighteen miles a second to accomplish one of its voyages round the sun in the lapse of 365-1/4 days. The question then arises as to whether the rate at which a planet moves is uniform or not. Does the earth, for instance, actually move at all times with the velocity of eighteen miles a second, or does our planet sometimes move more rapidly and sometimes more slowly, so that the average of eighteen miles a second is still maintained? This is a question of very great importance, and we are able to answer it in the clearest and most emphatic manner. The velocity of a planet is _not_ uniform, and the variations of that velocity can be explained by the adjoining figure (Fig. 38).
Let us first of all imagine the planet to be situated at that part of its path most distant from the sun towards the right of the figure. In this position the body's velocity is at its lowest; as the planet begins to approach the sun the speed gradually improves until it attains its mean value. After this point has been passed, and the planet is now rapidly hurrying on towards the sun, the velocity with which it moves becomes gradually greater and greater, until at length, as it dashes round the sun, its speed attains a maximum. After passing the sun, the distance of the planet from the luminary increases, and the velocity of the motion begins to abate; gradually it declines until the mean value is again reached, and then it falls still lower, until the body recedes to its greatest distance from the sun, by which time the velocity has abated to the value from which we supposed it to commence. We thus observe that the nearer the planet is to the sun the quicker it moves. We can, however, give numerical definiteness to the principle according to which the velocity of the planet varies. The adjoining figure (Fig. 39) shows a planetary orbit, with, of course, the sun at the focus S. We have taken two portions, A B and C D, round the ellipse, and joined their extremities to the focus. Kepler's second law may be stated in these words:--
"_Every planet moves round the sun with such a velocity at every
point, that a straight line drawn from it to the sun passes over
equal areas in equal times._"
For example, if the two shaded portions, A B S and D C S, are equal in area, then the times occupied by the planet in travelling over the portions of the ellipse, A B and C D, are equal. If the one area be greater than the other, then the times required are in the proportion of the areas.
This law being admitted, the reason of the increase in the planet's velocity when it approaches the sun is at once apparent. To accomplish a definite area when near the sun, a larger arc is obviously necessary than at other parts of the path. At the opposite extremity, a small arc suffices for a large area, and the velocity is accordingly less.
These two laws completely prescribe the motion of a planet round the sun. The first defines the path which the planet pursues; the second describes how the velocity of the body varies at different points along its path. But Kepler added to these a third law, which enables us to compare the movements of two different planets revolving round the same sun. Before stating this law, it is necessary to explain exactly what is meant by the _mean_ distance of a planet. In its elliptic path the distance from the sun to the planet is constantly changing; but it is nevertheless easy to attach a distinct meaning to that distance which is an average of all the distances. This average is called the mean distance. The simplest way of finding the mean distance is to add the greatest of these quantities to the
The curve which represents with perfect fidelity the movements of a planet in its revolution around the sun belongs to that well-known group of curves which mathematicians describe as the conic sections. The particular form of conic section which denotes the orbit of a planet is known by the name of the _ellipse_: it is spoken of somewhat less accurately as an oval. The ellipse is a curve which can be readily constructed. There is no simpler method of doing so than that which is familiar to draughtsmen, and which we shall here briefly describe.
We represent on the next page (Fig. 37) two pins passing through a sheet of paper. A loop of twine passes over the two pins in the manner here indicated, and is stretched by the point of a pencil. With a little care the pencil can be guided so as to keep the string stretched, and its point will then describe a curve completely round the pins, returning to the point from which it started. We thus produce that celebrated geometrical figure which is called an ellipse.
It will be instructive to draw a number of ellipses, varying in each case the circumstances under which they are formed. If, for instance, the pins remain placed as before, while the length of the loop is increased, so that the pencil is farther away from the pins, then it will be observed that the ellipse has lost some of its elongation, and approaches more closely to a circle. On the other hand, if the length of the cord in the loop be lessened, while the pins remain as before, the ellipse will be found more oval, or, as a mathematician would say, its _eccentricity_ is increased. It is also useful to study the changes which the form of the ellipse undergoes when one of the pins is altered, while the length of the loop remains unchanged. If the two pins be brought nearer together the eccentricity will decrease, and the ellipse will approximate more closely to the shape of a circle. If the pins be separated more widely the eccentricity of the ellipse will be increased. That the circle is an extreme form of ellipse will be evident, if we suppose the two pins to draw in so close together that they become coincident; the point will then simply trace out a circle as the pencil moves round the figure.
The points marked by the pins obviously possess very remarkable relations with respect to the curve. Each one is called a _focus_, and an ellipse can only have one pair of foci. In other words, there is but a single pair of positions possible for the two pins, when an ellipse of specified size, shape, and position is to be constructed.
The ellipse differs principally from a circle in the circumstance that it possesses variety of form. We can have large and small ellipses just as we can have large and small circles, but we can also have ellipses of greater or less eccentricity. If the ellipse has not the perfect simplicity of the circle it has, at least, the charm of variety which the circle has not. The oval curve has also the beauty derived from an outline of perfect grace and an association with ennobling conceptions.
The ancient geometricians had studied the ellipse: they had noticed its foci; they were acquainted with its geometrical relations; and thus Kepler was familiar with the ellipse at the time when he undertook his celebrated researches on the movements of the planets. He had found, as we have already indicated, that the movements of the planets could not be reconciled with circular orbits. What shape of orbit should next be tried? The ellipse was ready to hand, its properties were known, and the comparison could be made; memorable, indeed, was the consequence of this comparison. Kepler found that the movement of the planets could be explained, by supposing that the path in which each one revolved was an ellipse. This in itself was a discovery of the most commanding importance. On the one hand it reduced to order the movements of the great globes which circulate round the sun; while on the other, it took that beautiful class of curves which had exercised the geometrical talents of the ancients, and assigned to them the dignity of defining the highways of the universe.
But we have as yet only partly enunciated the first discovery of Kepler. We have seen that a planet revolves in an ellipse around the sun, and that the sun is, therefore, at some point in the interior of the ellipse--but at what point? Interesting, indeed, is the answer to this question. We have pointed out how the foci possess a geometrical significance which no other points enjoy. Kepler showed that the sun must be situated in one of the foci of the ellipse in which each planet revolves. We thus enunciate the first law of planetary motion in the following words:--
_Each planet revolves around the sun in an elliptic path, having
the sun at one of the foci._
We are now enabled to form a clear picture of the orbits of the planets, be they ever so numerous, as they revolve around the sun. In the first place, we observe that the ellipse is a plane curve; that is to say, each planet must, in the course of its long journey, confine its movements to one plane. Each planet has thus a certain plane appropriated to it. It is true that all these planes are very nearly coincident, at least in so far as the great planets are concerned; but still they are distinct, and the only feature in which they all agree is that each one of them passes through the sun. All the elliptic orbits of the planets have one focus in common, and that focus lies at the centre of the sun.
It is well to illustrate this remarkable law by considering the circumstances of two or three different planets. Take first the case of the earth, the path of which, though really an ellipse, is very nearly circular. In fact, if it were drawn accurately to scale on a sheet of paper, the difference between the elliptic orbit and the circle would hardly be detected without careful measurement. In the case of Venus the ellipse is still more nearly a circle, and the two foci of the ellipse are very nearly coincident with the centre of the circle. On the other hand, in the case of Mercury, we have an ellipse which departs from the circle to a very marked extent, while in the orbits of some of the minor planets the eccentricity is still greater. It is extremely remarkable that every planet, no matter how far from the sun, should be found to move in an ellipse of some shape or other. We shall presently show that necessity compels each planet to pursue an elliptic path, and that no other form of path is possible.
Started on its elliptic path, the planet pursues its stately course, and after a certain duration, known as the _periodic time_, regains the position from which its departure was taken. Again the planet traces out anew the same elliptic path, and thus, revolution after revolution, an identical track is traversed around the sun. Let us now attempt to follow the body in its course, and observe the history of its motion during the time requisite for the completion of one of its circuits. The dimensions of a planetary orbit are so stupendous that the planet must run its course very rapidly in order to finish the journey within the allotted time. The earth, as we have already seen, has to move eighteen miles a second to accomplish one of its voyages round the sun in the lapse of 365-1/4 days. The question then arises as to whether the rate at which a planet moves is uniform or not. Does the earth, for instance, actually move at all times with the velocity of eighteen miles a second, or does our planet sometimes move more rapidly and sometimes more slowly, so that the average of eighteen miles a second is still maintained? This is a question of very great importance, and we are able to answer it in the clearest and most emphatic manner. The velocity of a planet is _not_ uniform, and the variations of that velocity can be explained by the adjoining figure (Fig. 38).
Let us first of all imagine the planet to be situated at that part of its path most distant from the sun towards the right of the figure. In this position the body's velocity is at its lowest; as the planet begins to approach the sun the speed gradually improves until it attains its mean value. After this point has been passed, and the planet is now rapidly hurrying on towards the sun, the velocity with which it moves becomes gradually greater and greater, until at length, as it dashes round the sun, its speed attains a maximum. After passing the sun, the distance of the planet from the luminary increases, and the velocity of the motion begins to abate; gradually it declines until the mean value is again reached, and then it falls still lower, until the body recedes to its greatest distance from the sun, by which time the velocity has abated to the value from which we supposed it to commence. We thus observe that the nearer the planet is to the sun the quicker it moves. We can, however, give numerical definiteness to the principle according to which the velocity of the planet varies. The adjoining figure (Fig. 39) shows a planetary orbit, with, of course, the sun at the focus S. We have taken two portions, A B and C D, round the ellipse, and joined their extremities to the focus. Kepler's second law may be stated in these words:--
"_Every planet moves round the sun with such a velocity at every
point, that a straight line drawn from it to the sun passes over
equal areas in equal times._"
For example, if the two shaded portions, A B S and D C S, are equal in area, then the times occupied by the planet in travelling over the portions of the ellipse, A B and C D, are equal. If the one area be greater than the other, then the times required are in the proportion of the areas.
This law being admitted, the reason of the increase in the planet's velocity when it approaches the sun is at once apparent. To accomplish a definite area when near the sun, a larger arc is obviously necessary than at other parts of the path. At the opposite extremity, a small arc suffices for a large area, and the velocity is accordingly less.
These two laws completely prescribe the motion of a planet round the sun. The first defines the path which the planet pursues; the second describes how the velocity of the body varies at different points along its path. But Kepler added to these a third law, which enables us to compare the movements of two different planets revolving round the same sun. Before stating this law, it is necessary to explain exactly what is meant by the _mean_ distance of a planet. In its elliptic path the distance from the sun to the planet is constantly changing; but it is nevertheless easy to attach a distinct meaning to that distance which is an average of all the distances. This average is called the mean distance. The simplest way of finding the mean distance is to add the greatest of these quantities to the
Free e-book «The Story of the Heavens - Sir Robert Stawell Ball (ebook reader for laptop .TXT) 📗» - read online now
Similar e-books:
Comments (0)