The Story of the Heavens - Sir Robert Stawell Ball (ebook reader for laptop .TXT) 📗
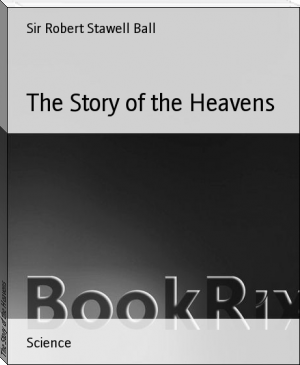
- Author: Sir Robert Stawell Ball
Book online «The Story of the Heavens - Sir Robert Stawell Ball (ebook reader for laptop .TXT) 📗». Author Sir Robert Stawell Ball
If we take our earth itself, conceive it comminuted into dust, and allow that dust to fall on the sun as a mighty shower, each fragment would instantly give out a quantity of heat, and the whole would add to the sun a supply of heat adequate to sustain the present rate of radiation for nearly one hundred years. The mighty mass of Jupiter treated in the same way would generate a meteoric display greater in the ratio in which the mass of Jupiter exceeds the mass of earth. Were Jupiter to fall into the sun, enough heat would be thereby produced to scorch the whole solar system; while all the planets together would be capable of producing heat which, if properly economised, would supply the radiation of the sun for 45,000 years.
It must be remembered that though the moon could supply one year's heat, and Jupiter 30,000 years' heat, yet the practical question is not whether the solar system could supply the sun's heat, but whether it does. Is it likely that meteors equal in mass to the moon fall into the sun every year? This is the real question, and I think we are bound to reply to it in the negative. It can be shown that the quantity of meteors which could be caught by the sun in any one year can be only an excessively minute fraction of the total amount. If, therefore, a moon-weight of meteors were caught every year, there must be an incredible mass of meteoric matter roaming at large through the system. There must be so many meteors that the earth would be incessantly pelted with them, and heated to such a degree as to be rendered uninhabitable. There are also other reasons which preclude the supposition that a stupendous quantity of meteoric matter exists in the vicinity of the sun. Such matter would produce an appreciable effect on the movement of the planet Mercury. There are, no doubt, some irregularities in the movements of Mercury not yet fully explained, but these irregularities are very much less than would be the case if meteoric matter existed in quantity adequate to the sustentation of the sun. Astronomers, then, believe that though meteors may provide a rate in aid of the sun's current expenditure, yet that the greater portion of that expenditure must be defrayed from other resources.
It is one of the achievements of modern science to have effected the solution of the problem--to have shown how it is that, notwithstanding the stupendous radiation, the sun still maintains its temperature. The question is not free from difficulty in its exposition, but the matter is one of such very great importance that we are compelled to make the attempt.
Let us imagine a vast globe of heated gas in space. This is not an entirely gratuitous supposition, inasmuch as there are globes apparently of this character; they have been already alluded to as planetary nebulae. This globe will radiate heat, and we shall suppose that it emits more heat than it receives from the radiation of other bodies. The globe will accordingly lose heat, or what is equivalent thereto, but it will be incorrect to assume that the globe will necessarily fall in temperature. That the contrary is, indeed, the case is a result almost paradoxical at the first glance; but yet it can be shown to be a necessary consequence of the laws of heat and of gases.
Let us fix our attention on a portion of the gas lying on the surface of the globe. This is, of course, attracted by all the rest of the globe, and thus tends in towards the centre of the globe. If equilibrium subsists, this tendency must be neutralised by the pressure of the gas beneath; so that the greater the gravitation, the greater is the pressure. When the globe of gas loses heat by radiation, let us suppose that it grows colder--that its temperature accordingly falls; then, since the pressure of a gas decreases when the temperature falls, the pressure beneath the superficial layer of the gas will decrease, while the gravitation is unaltered. The consequence will inevitably be that the gravitation will now conquer the pressure, and the globe of gas will accordingly contract. There is, however, another way in which we can look at the matter. We know that heat is equivalent to energy, so that when the globe radiates forth heat, it must expend energy. A part of the energy of the globe will be due to its temperature; but another, and in some respects a more important, part is that due to the separation of its particles. If we allow the particles to come closer together we shall diminish the energy due to separation, and the energy thus set free can take the form of heat. But this drawing in of the particles necessarily involves a shrinking of the globe.
And now for the remarkable consequence, which seems to have a very important application in astronomy. As the globe contracts, a part of its energy of separation is changed into heat; that heat is partly radiated away, but not so rapidly as it is produced by the contraction. The consequence is, that although the globe is really losing heat and really contracting, yet that its temperature is actually rising.[43] A simple case will suffice to demonstrate this result, paradoxical as it may at first seem. Let us suppose that by contraction of the sphere it had diminished to one-half its diameter; and let us fix our attention on a cubic inch of the gaseous matter in any point of the mass. After the contraction has taken place each edge of the cube would be reduced to half an inch, and the volume would therefore be reduced to one-eighth part of its original amount. The law of gases tells us that if the temperature be unaltered the pressure varies inversely as the volume, and consequently the internal pressure in the cube would in that case be increased eightfold. As, however, in the case before us, the distance between every two particles is reduced to one-half, it will follow that the gravitation between every two particles is increased fourfold, and as the area is also reduced to one-fourth, it will follow that the pressure inside the reduced cube is increased sixteenfold; but we have already seen that with a constant temperature it only increases eightfold, and hence the temperature cannot be constant, but must rise with the contraction.
We thus have the somewhat astonishing result that a gaseous globe in space radiating heat, and thereby growing smaller, is all the time actually increasing in temperature. But, it may be said, surely this cannot go on for ever. Are we to suppose that the gaseous mass will go on contracting and contracting with a temperature ever fiercer and fiercer, and actually radiating out more and more heat the more it loses? Where lies the limit to such a prospect? As the body contracts, its density must increase, until it either becomes a liquid, or a solid, or, at any rate, until it ceases to obey the laws of a purely gaseous body which we have supposed. Once these laws cease to be observed the argument disappears; the loss of heat may then really be attended with a loss of temperature, until in the course of time the body has sunk to the temperature of space itself.
It is not assumed that this reasoning can be applied in all its completeness to the present state of the sun. The sun's density is now so great that the laws of gases cannot be there strictly followed. There is, however, good reason to believe that the sun was once more gaseous than at present; possibly at one time he may have been quite gaseous enough to admit of this reasoning in all its fulness. At present the sun appears to be in some intermediate stage of its progress from the gaseous condition to the solid condition. We cannot, therefore, say that the temperature of the sun is now increasing in correspondence with the process of contraction. This may be true or it may not be true; we have no means of deciding the point. We may, however, feel certain that the sun is still sufficiently gaseous to experience in some degree the rise of temperature associated with the contraction. That rise in temperature may be partly or wholly obscured by the fall in temperature which would be the more obvious consequence of the radiation of heat from the partially solid body. It will, however, be manifest that the cooling of the sun may be enormously protracted if the fall of temperature from the one cause be nearly compensated by the rise of temperature from the other. It can hardly be doubted that in this we find the real explanation of the fact that we have no historical evidence of any appreciable alteration in the radiation of heat from the sun.
This question is one of such interest that it may be worth while to look at it from a slightly different point of view. The sun contains a certain store of energy, part of which is continually disappearing in the form of radiant heat. The energy remaining in the sun is partly transformed in character; some of it is transformed into heat, which goes wholly or partly to supply the loss by radiation. The total energy of the sun must, however, be decreasing; and hence it would seem the sun must at some time or other have its energy exhausted, and cease to be a source of light and of heat. It is true that the rate at which the sun contracts is very slow. We are, indeed, not able to measure with certainty the decrease in the sun's bulk. It is a quantity so minute, that the contraction since the birth of accurate astronomy is not large enough to be perceptible in our telescopes. It is, however, possible to compute what the contraction of the sun's bulk must be, on the supposition that the energy lost by that contraction just suffices to supply the daily radiation of heat. The change is very small when we consider the present size of the sun. At the present time the sun's diameter is about 860,000 miles. If each year this diameter decreases by about 300 feet, sufficient energy will be yielded to account for the entire radiation. This gradual decrease is always in progress.
These considerations are of considerable interest when we apply them retrospectively. If it be true that the sun is at this moment shrinking, then in past times his globe must have been greater than it is at present. Assuming the figures already given, it follows that one hundred years ago the diameter of the sun must have been nearly six miles greater than it is now; one thousand years ago the diameter was fifty-seven miles greater; ten thousand years ago the diameter of the sun was five hundred and seventy miles greater than it is to-day. When man first trod this earth it would seem that the sun must have been many hundreds, perhaps many thousands, of miles greater than it is at this time.
We must not, however, over-estimate the significance of this statement. The diameter of the sun is so great, that a diminution of 10,000 miles would be but little more than the hundredth part of its diameter. If it were suddenly to shrink to the extent of 10,000 miles, the change would not be appreciable to ordinary observation, though a much smaller change would not elude delicate astronomical measurement. It does not necessarily follow that the climates on our earth in these early times must have been very different from those which we find at this day, for the question of climate depends upon other matters besides sunbeams.
Yet
It must be remembered that though the moon could supply one year's heat, and Jupiter 30,000 years' heat, yet the practical question is not whether the solar system could supply the sun's heat, but whether it does. Is it likely that meteors equal in mass to the moon fall into the sun every year? This is the real question, and I think we are bound to reply to it in the negative. It can be shown that the quantity of meteors which could be caught by the sun in any one year can be only an excessively minute fraction of the total amount. If, therefore, a moon-weight of meteors were caught every year, there must be an incredible mass of meteoric matter roaming at large through the system. There must be so many meteors that the earth would be incessantly pelted with them, and heated to such a degree as to be rendered uninhabitable. There are also other reasons which preclude the supposition that a stupendous quantity of meteoric matter exists in the vicinity of the sun. Such matter would produce an appreciable effect on the movement of the planet Mercury. There are, no doubt, some irregularities in the movements of Mercury not yet fully explained, but these irregularities are very much less than would be the case if meteoric matter existed in quantity adequate to the sustentation of the sun. Astronomers, then, believe that though meteors may provide a rate in aid of the sun's current expenditure, yet that the greater portion of that expenditure must be defrayed from other resources.
It is one of the achievements of modern science to have effected the solution of the problem--to have shown how it is that, notwithstanding the stupendous radiation, the sun still maintains its temperature. The question is not free from difficulty in its exposition, but the matter is one of such very great importance that we are compelled to make the attempt.
Let us imagine a vast globe of heated gas in space. This is not an entirely gratuitous supposition, inasmuch as there are globes apparently of this character; they have been already alluded to as planetary nebulae. This globe will radiate heat, and we shall suppose that it emits more heat than it receives from the radiation of other bodies. The globe will accordingly lose heat, or what is equivalent thereto, but it will be incorrect to assume that the globe will necessarily fall in temperature. That the contrary is, indeed, the case is a result almost paradoxical at the first glance; but yet it can be shown to be a necessary consequence of the laws of heat and of gases.
Let us fix our attention on a portion of the gas lying on the surface of the globe. This is, of course, attracted by all the rest of the globe, and thus tends in towards the centre of the globe. If equilibrium subsists, this tendency must be neutralised by the pressure of the gas beneath; so that the greater the gravitation, the greater is the pressure. When the globe of gas loses heat by radiation, let us suppose that it grows colder--that its temperature accordingly falls; then, since the pressure of a gas decreases when the temperature falls, the pressure beneath the superficial layer of the gas will decrease, while the gravitation is unaltered. The consequence will inevitably be that the gravitation will now conquer the pressure, and the globe of gas will accordingly contract. There is, however, another way in which we can look at the matter. We know that heat is equivalent to energy, so that when the globe radiates forth heat, it must expend energy. A part of the energy of the globe will be due to its temperature; but another, and in some respects a more important, part is that due to the separation of its particles. If we allow the particles to come closer together we shall diminish the energy due to separation, and the energy thus set free can take the form of heat. But this drawing in of the particles necessarily involves a shrinking of the globe.
And now for the remarkable consequence, which seems to have a very important application in astronomy. As the globe contracts, a part of its energy of separation is changed into heat; that heat is partly radiated away, but not so rapidly as it is produced by the contraction. The consequence is, that although the globe is really losing heat and really contracting, yet that its temperature is actually rising.[43] A simple case will suffice to demonstrate this result, paradoxical as it may at first seem. Let us suppose that by contraction of the sphere it had diminished to one-half its diameter; and let us fix our attention on a cubic inch of the gaseous matter in any point of the mass. After the contraction has taken place each edge of the cube would be reduced to half an inch, and the volume would therefore be reduced to one-eighth part of its original amount. The law of gases tells us that if the temperature be unaltered the pressure varies inversely as the volume, and consequently the internal pressure in the cube would in that case be increased eightfold. As, however, in the case before us, the distance between every two particles is reduced to one-half, it will follow that the gravitation between every two particles is increased fourfold, and as the area is also reduced to one-fourth, it will follow that the pressure inside the reduced cube is increased sixteenfold; but we have already seen that with a constant temperature it only increases eightfold, and hence the temperature cannot be constant, but must rise with the contraction.
We thus have the somewhat astonishing result that a gaseous globe in space radiating heat, and thereby growing smaller, is all the time actually increasing in temperature. But, it may be said, surely this cannot go on for ever. Are we to suppose that the gaseous mass will go on contracting and contracting with a temperature ever fiercer and fiercer, and actually radiating out more and more heat the more it loses? Where lies the limit to such a prospect? As the body contracts, its density must increase, until it either becomes a liquid, or a solid, or, at any rate, until it ceases to obey the laws of a purely gaseous body which we have supposed. Once these laws cease to be observed the argument disappears; the loss of heat may then really be attended with a loss of temperature, until in the course of time the body has sunk to the temperature of space itself.
It is not assumed that this reasoning can be applied in all its completeness to the present state of the sun. The sun's density is now so great that the laws of gases cannot be there strictly followed. There is, however, good reason to believe that the sun was once more gaseous than at present; possibly at one time he may have been quite gaseous enough to admit of this reasoning in all its fulness. At present the sun appears to be in some intermediate stage of its progress from the gaseous condition to the solid condition. We cannot, therefore, say that the temperature of the sun is now increasing in correspondence with the process of contraction. This may be true or it may not be true; we have no means of deciding the point. We may, however, feel certain that the sun is still sufficiently gaseous to experience in some degree the rise of temperature associated with the contraction. That rise in temperature may be partly or wholly obscured by the fall in temperature which would be the more obvious consequence of the radiation of heat from the partially solid body. It will, however, be manifest that the cooling of the sun may be enormously protracted if the fall of temperature from the one cause be nearly compensated by the rise of temperature from the other. It can hardly be doubted that in this we find the real explanation of the fact that we have no historical evidence of any appreciable alteration in the radiation of heat from the sun.
This question is one of such interest that it may be worth while to look at it from a slightly different point of view. The sun contains a certain store of energy, part of which is continually disappearing in the form of radiant heat. The energy remaining in the sun is partly transformed in character; some of it is transformed into heat, which goes wholly or partly to supply the loss by radiation. The total energy of the sun must, however, be decreasing; and hence it would seem the sun must at some time or other have its energy exhausted, and cease to be a source of light and of heat. It is true that the rate at which the sun contracts is very slow. We are, indeed, not able to measure with certainty the decrease in the sun's bulk. It is a quantity so minute, that the contraction since the birth of accurate astronomy is not large enough to be perceptible in our telescopes. It is, however, possible to compute what the contraction of the sun's bulk must be, on the supposition that the energy lost by that contraction just suffices to supply the daily radiation of heat. The change is very small when we consider the present size of the sun. At the present time the sun's diameter is about 860,000 miles. If each year this diameter decreases by about 300 feet, sufficient energy will be yielded to account for the entire radiation. This gradual decrease is always in progress.
These considerations are of considerable interest when we apply them retrospectively. If it be true that the sun is at this moment shrinking, then in past times his globe must have been greater than it is at present. Assuming the figures already given, it follows that one hundred years ago the diameter of the sun must have been nearly six miles greater than it is now; one thousand years ago the diameter was fifty-seven miles greater; ten thousand years ago the diameter of the sun was five hundred and seventy miles greater than it is to-day. When man first trod this earth it would seem that the sun must have been many hundreds, perhaps many thousands, of miles greater than it is at this time.
We must not, however, over-estimate the significance of this statement. The diameter of the sun is so great, that a diminution of 10,000 miles would be but little more than the hundredth part of its diameter. If it were suddenly to shrink to the extent of 10,000 miles, the change would not be appreciable to ordinary observation, though a much smaller change would not elude delicate astronomical measurement. It does not necessarily follow that the climates on our earth in these early times must have been very different from those which we find at this day, for the question of climate depends upon other matters besides sunbeams.
Yet
Free e-book «The Story of the Heavens - Sir Robert Stawell Ball (ebook reader for laptop .TXT) 📗» - read online now
Similar e-books:
Comments (0)